The development of the microscope in the 17th century magnified our awareness of a microbial universe previously invisible to the naked eye. Anton van Leeuwenhoek, a Dutch textile draper and science hobbyist, was one of the first individuals to glance into the microbial looking glass and identify unicellular organisms (so-called animalcules) such as protozoa and bacteria [1]. His colleague, Robert Hooke, went on to publish the seminal text, Micrographia, which described his observations of microfungi [2]. Two centuries later, Louis Pasteur validated the role of microbes in fermentation. However, Pasteur also gave weight to Ignaz Semmelweis’ controversial germ theory of disease stating that microbes have the capacity to cause pathological effects on our human health [3].
These early studies in microbiology have provided significant insight on the human-microbe interaction characterized by either mutually beneficial or lethal outcomes. The duality of microbial behavior has dramatically impacted our perception of these microorganisms. Our cultural germophobia often precludes our ability to recognize the naturally transformative and symbiotic properties of microbes from the fermentation of grape juice into wine to their invaluable role in human digestion.
Michael Pollan, author of Cooked, explores a myriad of cooking traditions including those that directly involve the action of microbes such as bacteria and yeast. In his depiction of ancient sourdough recipes, he lists four ingredients: whole grain flour, water, salt, and the repertoire of microbes in the air [4]. The microbes catalyze slow-fermentation reactions (taking up to 24 hours) that leaven the bread while transforming molecules into digestible nutrients for us to absorb.
Whether we can stomach it or not, we all possess unique microbial signatures that are composed of trillions of microorganisms living both inside our bodies and on the surface of our skin [5]. These microbial communities, referred to as the human microbiome, cohabitate in our various mucosal, gastrointestinal, and epidermal surfaces. Symbiotic microbes are tolerated by our immune system and work collaboratively with our own bodies to digest the foods that we eat at the molecular level.
Our evolutionary history with bacteria is fueled by the currency of nutrition. In other words, our diet has a significant impact on the composition of our gut microbiota. Food consumption habits can either encourage the intestinal bloom of beneficial bacteria or opportunistic, disease-causing bacteria. Certain foods contain vital prebiotic molecules that encourage the expansion of beneficial bacterial species in the gut. High fiber foods including whole grains (brown rice, oats), vegetables (broccoli, peas) and legumes (lentils, black beans) contain an invaluable source of metabolic substrates that are converted into short-chain fatty acids by bacteria [6]. These short-chain fatty acids, such as butyrate, help to propagate microflora such as Bifidobacterium and Lactobacillus and maintain gastrointestinal tissue health [7].
If we were to design a perfect microbial habitat–a Gutopia, if you will, it would be a homeostatic organ city of diverse symbiotic microbiota that is rich in fiber economy and free of any harmful pollutants. However, our dietary choices can tip the balance of this intestinal paradise and create a dystopic environment suitable for the expansion of pathogenic microbes.
Contemporary eating habits that are characteristically high in fat and carbohydrates are responsible for the emergence of modern diseases such as diabetes, colorectal cancer, and inflammatory bowel diseases [8-11]. Recent studies suggest that compositional changes in the intestinal microbiome can encourage the bloom of disease-causing microflora. The dramatic alteration of today’s eating behavior introduces gastrointestinal disturbances or challenges that our bodies and microbial counterparts have not evolved to accommodate. For example, a study investigating the consumption of different dietary fats in immunocompromised IL-10–/– mice identified the expansion of B. wadsworthia; a gram-negative, sulfur-reducing species of bacteria flourished in mice fed a diet high in saturated fat , but not in low-fat or polyunsaturated fat diets [12-13]. The bloom of B. wadsworthia was attributed to the unregulated production of a bile salt known as taurocholic acid brought about by the overconsumption of saturated fat.
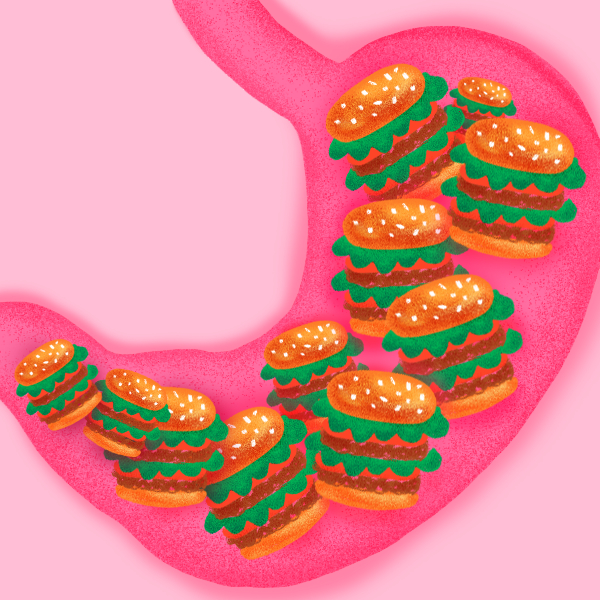
Imbalanced dietary intake and overconsumption of foods high in saturated fats can impact the ecology of gut microbiota [Illustration by Grace Danico]
Taurocholic acid is an important source of sulfur that can stimulate the growth and maintenance of the pathogenic B. wadsworthia in these mice. The presence of this bacterium activates murine proinflammatory immune defense mechanisms that compromise the permeability of gut mucosal tissue causing intestinal inflammation. This pathogenic etiology of gut inflammation is implicated in the onset of Crohn’s disease and ulcerative colitis [12-13].
Much like any city there are complications that arise, which can tip the balance between utopic and dystopic environments. In the case of our gut health, we should imagine ourselves as landscape architects cultivating the balanced ecology of our microbiome. If we feed our intestinal gardens with the right balance of foods we can foster the growth of symbiotic bacteria while discouraging the bloom of pestilent, pathogenic microbial weeds.
The human microbiome not only affects our gut health but can have some profound effects on our behavior and brain function. Dr. Elaine Hsiao, Assistant Professor in the Department of Integrative Biology and Physiology at UCLA, investigates the interplay between our commensal microbes and their role in neurological development and function. Her work has linked the perturbations in gut microbiota with the onset of neurological disorders such as autism [14].
As we continue to study the ecology and diversity of microbes living within and around us, we are faced with many fundamental challenges in testing the dynamics of these microbial communities. From a clinical perspective, physicians and researchers alike have utilized human fecal samples to identify unique microbial gut profiles in their patients. These samples serve as powerful investigative tools in our pursuit to understand how certain commensal microbes can cause or serve as diagnostic readouts. The power of the sequencing technology used to characterize microbes in stool samples (16s RNA sequencing) comes from its level of coverage-the ability to identify the majority of bacteria in a sample. However, sequencing depth-the resolution at which a species can be identified remains challenging. Additionally, many microbial species have been challenging to culture in vitro, making it difficult for researchers to repeatedly perform experiments in a laboratory setting and gain a deeper mechanistic understanding of microbial behavior and ecology.
Dr. Rachel Dutton, Assistant Professor in the Division of Biological Sciences at UCSD, addresses some of these technological limitations by studying the establishment and maintenance of microbial communities in different types of cheeses. With this model, her lab can investigate the interactions of different types of microbes to better understand them as ecological systems [15-16].
Science & Food is honored to host Elaine Hsiao and Rachel Dutton for the 2016 UCLA Science & Food public lecture series to elaborate on their findings. They will be accompanied by Sander Katz, author of Wild Fermentation, who will discuss the transformative properties of microbes in the production of foods like sauerkraut.
Join us on Wednesday, May 11th at 7PM in Schoenberg Hall at UCLA for “Microbes: From Your Food to Your Brain” to learn more about the intriguing world of microbes!
References cited
- Gest H. “The discovery of microorganisms by Robert Hooke and Antoni Van Leeuwenhoek, fellows of the Royal Society”. Notes Rec R Soc Lond. 5 (2004). 187-201.
- Hooke R. “Micrographia” Jo. Martyn & Ja. Allestry (1665).
- “The History of the Germ Theory” The British Medical Journal. 1 (1888).
- Pollan M. Cooked: A Natural History of Transformation. Penguin Books. (2013).
- Abbott A. “Scientist bust myth that our bodies have more bacteria than human cells” Nature. (2016).
- Leone V, Chang EB, Devkota SD. “Diet, microbes, and host genetics: the perfect storm in inflammatory bowel disease” J. Gastroenterol 48 (2013). 315-321.
- Sartor RB,.“Microbial influences in inflammatory bowel disease: role in pathogenesis and clinical implications” Elsevier (2004). 138-162.
- Hotamisligil, GS. “Inflammation and metabolic disorders” Nature. 444 (2006). 860-867.
- Parkin DM, Bray F, Ferlay J, et al. “Global cancer statistics” CA Cancer J Clin. 55. (2005). 74-108.
- Loftus EV Jr. “Clinical epidemiology of inflammatory bowel disease: incidence, prevalence, and environmental influences” Gastroenterology 126. (2004). 1504-1517.
- Molodecky NA, Soon IS, Rabi EM, et al. “Increasing incidence and prevalence of the inflammatory bowel diseases with time, based on systemic review” Gastroenterology 142. (2012). 46-54.
- Devkota SD, Wang Y, Musch MW, et al. “Dietary-fat-induced taurocholic acid promotes pathobiont expansion and colitis in Il10-/- mice” Nature. 487 (2012). 104-108.
- Devkota SD, Chang EB. “Diet-induced expansion of pathobionts in experimental colitis” Gut Microbes. 4:2 (2013). 172-174.
- Hsiao E.Y., “Gastrointestinal issues in autism spectrum disorder”, Harv Rev Psychiatry, 22 (2014). 104-111.
- Wolfe BE, Dutton RJ. “Fermented Foods as Experimentally Tractable Microbial Ecosystems” Cell. 161(1) (2015). 49-55.
- Wolfe BE, Button JE, Sanarelli M, Dutton RJ. “Cheese rind communities provide tractable systems for in situ and in vitro studies of microbial diversity” Cell. 158 (2014). 422-433.
About the author: Anthony Martin received his Ph.D. in Genetic, Cellular and Molecular Biology at USC and is self-publishing a cookbook of his favorite Filipino dishes.
Read more by Anthony Martin