Engineering the Perfect Gingerbread House
Building gingerbread houses can be a frustrating process. An idyllic three-story building with crystal sugar snow, marshmallow snowmen, and gumdrop twinkle lights can quickly end in a collapsed mess, sending icing windows and candy cane gates into disarray. But don’t resort to those trusty milk cartons and graham crackers just yet – with a few changes in technique, your dream house can easily be obtained.
During our recent “Engineering the Perfect Gingerbread House” event, graduate student Kendra Nyberg taught UCLA students about the best practices for gingerbread construction. Her lecture delved into the molecular makeup of the materials and the physics behind the structure.
Base Construction Materials: Gingerbread and Icing
Gingerbread should be sturdy and demonstrate elasticity, which is the measure of its ability to resist deformation [1]. Because the gingerbread walls will be under stress from the roof, there needs to be sufficient resistance to avoid cracking or total collapse. Dough with a tough, springy consistency and decreased moisture content is ideal, and can be achieved by using flour with high protein content, such as bread flour. Higher-protein flours contain more glutenin and gliadin proteins, which create the springy gluten network that gives dough its elastic properties.
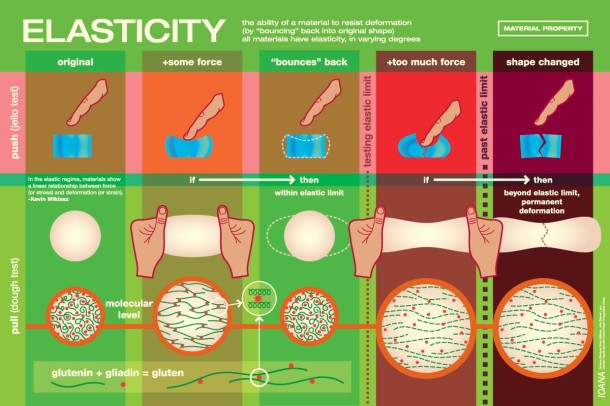
Photo credit: Ionacolor.com
Icing serves as the glue that holds the entire structure together. The mixture should be just pliable enough to hold the gingerbread pieces together before drying into a hard, unmovable substance. Here egg whites are key. When beaten, the egg’s proteins denature and then coagulate, stabilizing air bubbles in the icing and creating white, foamy “peaks” that vary in their stiffness and resistance to gravity. Stiffer peaks are better for gingerbread icing, and more coagulated proteins can contribute to a stronger paste.

Photo credit: Advanced Materials
Why use icing instead of frosting? Both confections contain copious amounts of sugar, but where icing contains egg whites, frosting typically incorporates butter. The additional fat globules from butter provide some thickness and stability to the frosting. However, since standard buttercream frosting does not contain egg whites, the only proteins present are those from the milk in the form of butter. Although these proteins are perfect for dense and creamy cupcake topping, they do not assemble into the stiff, strong networks needed for gingerbread house construction.
Stability and Height: Architectural design
Once the bricks and mortar of your gingerbread house have been created, you can move onto the creative part of the process – construction. There are many forces acting on a gingerbread house. Consider the roof: forces on the sloping gingerbread roof includes friction from the frosting, a normal force perpendicular to the gingerbread surface, and gravity pulling the roof toward the floor. These forces also show up to varying degrees in all of the upright walls of the gingerbread house. To avoid collapse, it is best to spread out the forces over many surfaces. For example, a wider structure with a flatter rooftop will be sturdier than a narrow house with a sloping roof.

Normal, friction, and gravity forces acting on a gingerbread roof
Photo credit: dallassd.com
If the height of the house is very high, the gingerbread is also more sensitive to buckling under the added weight of the extra gingerbread. To prevent buckling, you can calculate the critical height at which buckling occurs, which depends on such factors as gingerbread density and the force of gravity [2].

Photo credit: Tim Jones (Zoonomian)
You don’t have to be an engineer or an architect to construct the perfect gingerbread house. With the proper dough, frosting, and design considerations, the house of your dreams can be achieved – perfect to last as a display through the holidays. Now get building!
References cited
- Iona (2011) “Elasticity (TV set art) (3).” blog.ionacolor.com
- Tim Jones (2013) “Musings on Structural Gingerbread.”
About the author: Catherine Hu is pursuing her B.S. in Psychobiology at UCLA. When she is not writing about food science, she enjoys exploring the city and can often be found enduring long wait times to try new mouthwatering dishes.