Sauerkraut
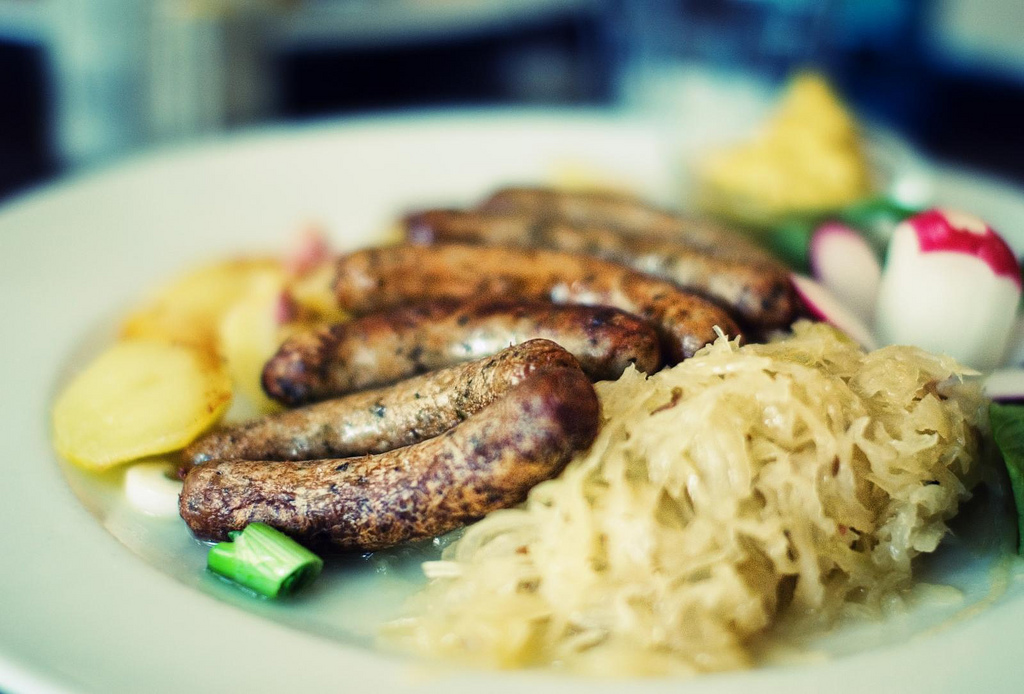
Photo credit: Anders Adermark (cmbellman/Flickr)
Fizzy, bitter, yeasty, sour, floral, and sometimes just downright offensive—there are a dazzling array of adjectives that can come to mind when you think of fermentation. Fermentation is one of world’s oldest and simplest culinary traditions. Serendipitously discovered in ancient times as a means of preservation, flavor enhancement, and intoxication, it has exploded as an art and scientific field in recent years. Aptly described by Chef David Chang as when “rotten goes right,” fermentation is a process that harnesses the power of benign microbes to produce complex flavors and can transform a seemingly rotten pile of vegetables into a curiously palatable delight (1). It’s fermentation we can thank for transforming a lonely, simple cabbage into a bustling hotspot for microbial activity that we know as sauerkraut.
German for “sour cabbage,” sauerkraut is distinctively tangy, floral, and surprisingly simple to create. It requires no specialized ingredients or starters, demanding just cabbage and salt (1). Sauerkraut is an example of wild fermentation, a process that exploits microbes native to the surface of cabbages. To make a batch, begin with finely shredded cabbage with roughly 3 tbsp salt per 5 lbs of cabbage. Give your fingers a workout by gently massaging the cabbage mix and after a few minutes, you’ll notice that the cabbage appears to be sweating (2). This sweat is the basis for our brine, whose presence is absolutely vital in our cabbage-to-sauerkraut transformation.
This brine sets a stage for successful sauerkraut fermentation, a fermentation made possible by the flavorful collaboration between several different microbes. These microbes, specifically lactic acid bacteria and yeast, thrive in salty, anaerobic environments, much like in the brine (1). Although the brine is simple, comprising just salt and water, it must be carefully controlled so as to provide the lactic acid bacteria and yeasts with a competitive advantage over other undesirable organisms. Too much salt and you ruin the palatability of our sauerkraut, but too little salt and you risk creating an environment favorable to spoilage or pathogenic bacteria (3).
As the microbes feed on sugars in cabbage, they mainly produce lactic acid, which like salt lends taste in addition to antimicrobial effects. As the brine becomes enriched with lactic acid, the pH declines and the sauerkraut begins to develop tart notes. These effects inhibit the growth of our unwanted microbes, which tend to be sensitive to acidity. Secondary products can also include carbon dioxide, alcohol, and acetic acid, all of which can suppress the growth of our unwanted organisms, too (3).
After massaging long enough, you should have enough brine to prepare your cabbage for jarring. Employing a willing and eager fist, pack your cabbage into a jar so that it’s completely submerged beneath the recently-created brine—you may also want to consider weighing it down. Ensure that your cabbage is entirely covered by brine, otherwise you risk inviting the growth of harmful aerobic bacteria into your jar. Anything left exposed to air is susceptible to mold or invasion of other organisms (2).
Depending on your preferences, you can let your sauerkraut ferment for just a few days or up to several weeks if you prefer stronger flavors. Leave it in a closet, on your countertop, or bury it in your backyard—it’s totally up to you!
References cited
- McGee, Harold. On food and cooking: the science and lore of the kitchen. New York: Scribner, 2004. Print.
- Katz, Sandor E. Wild Fermentation: The Flavor, Nutrition, and Craft of Live-Culture Foods. White River Junction, VT: Chelsea Green Pub, 2003. Print.
- Katz, Sandor E., Michael Pollan. The Art of Fermentation: An In-Depth Exploration of Essential Concepts and Processes from Around the World. White River Junction, VT: Chelsea Green Pub, 2012. Print.
About the author: Mai Nguyen is an aspiring food scientist who received her B.S. in biochemistry from the University of Virginia. She hopes to soon escape the bench in pursuit of a more creative and fulfilling career.